Introduction
Mechanical refrigeration is a thermodynamic process of removing heat
from a lower temperature heat source or substance and transferring it to a
higher temperature heat sink. This is against the Second Law of
Thermodynamics, which states that heat will not pass from a cold region to a warm one.
According to Clausius Statement of the Second Law of thermodynamics "It is
impossible to construct a device that operates in a cycle and produces no effect
other than the transfer of heat from a lower-temperature body to a higher-temperature
body ' Therefore in order to accomplish the transfer of heat from low
temperature region to high temperature region an "external agent" or energy
Input is required and you need a device, like a heat pump or refrigerator, which consumes
work. The operating principle of the refrigeration cycle was described
mathematically by Sadi Carnot in 1824 as a heat engine. A refrigerator or heat pump is simply
a heat engine operating in reverse. Note the direction of arrows: Heat engine
is defined as a device that converts heat energy into mechanical energy whereas the
heat pump or refrigerator is defined as a device that use mechanical energy to
pump heat from cold to hot region. A refrigeration system is a combination of
components and equipment connected in a sequential order to produce the refrigeration
effect. The most common refrigeration system in use today involves the input of
work (from a compressor) and uses the Vapor Compression Cycle. This course is an
overview of vapor compression refrigeration cycle, principles of heat generation,
transfer and rejection. Since refrigeration deals entirely with the removal or
transfer of heat, some knowledge of the nature and effects of heat is necessary for a
clear understanding of the subject. You may refer to the basic
thermodynamics and glossary of terms at the end to
help you with this Section first time through.
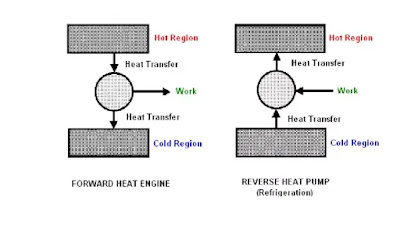
Air cycle refrigeration systems belong to the general class of gas cycle refrigeration systems, in which a gas is used as the working fluid.
The gas does not undergo any phase change during the cycle, consequently, all the
internal heat transfer processes are sensible heat transfer processes. Gas cycle
refrigeration systems find applications
refrigeration system is a combination of components and equipment connected in a sequential order to produce the refrigeration
effect. The most common refrigeration system in use today involves the input of
work (from a compressor) and uses the Vapor Compression Cycle. This course is an
overview of vapor compression refrigeration cycle, principles of heat generation,
transfer and rejection. Since refrigeration deals entirely with the removal or
transfer of heat, some knowledge of the nature and effects of heat is necessary for a
clear understanding of the subject. You may refer to the basic
thermodynamics and glossary of terms at the end to
help you with this Section first time through. Air cycle refrigeration systems belong to the general class of gas
cycle refrigeration systems, in which a gas is used as the working fluid. The gas does not
undergo any phase change during the cycle, consequently, all the internal heat transfer
processes are sensible heat transfer processes. Gas cycle refrigeration systems find
applications in air craft cabin cooling and also in the liquefaction of various gases. In the present
chapter gas cycle refrigeration systems based on air are discussed.
Reversed Carnot cycle employing a gas
Reversed Carnot cycle is an ideal refrigeration cycle for constant
temperature external heat source and heat sinks. Figure 9.1 (a) shows the schematic of a
reversed Carnot refrigeration system using a gas as the working fluid along with the cycle diagram
on T-s and P-v
coordinates. As shown, the cycle consists of the following four
processes:
Process 1-2: Reversible, adiabatic compression in a compressor
Process 2-3: Reversible, isothermal heat rejection in a compressor
Process 3-4: Reversible adiabatic expansion in a turbine
Process 4-1 : Reversible, isothermal heat absorption in a turbine
Schematic of a reverse Carnot
refrigeration system
Temperature Limitations of Carnot cycle:
Carnot cycle is an idealization and it suffers from several practical
limitations. One of the main difficulties with Carnot cycle employing a gas is the difficulty
of achieving isothermal heat transfer during processes 2-3 and 4-1. For a gas to have
heat transfer isothermally, it is essential to carry out work transfer from or to
the system when heat is transferred to the system (process 4-1) or from the system (process
2-3). This is difficult to achieve in practice. In addition, the volumetric refrigeration
capacity of the Carnot system is very small leading to large compressor displacement, which gives
rise to large frictional effects. All actual processes are irreversible, hence completely
reversible cycles are idealizations only.
BELL COLEMAN CYCLE
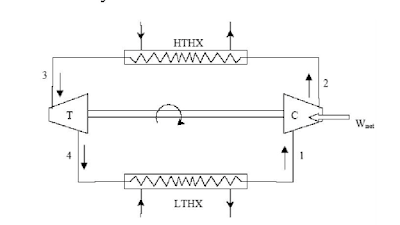 |
Schematic of a closed reverse
Brayton cycle This is an important cycle frequently employed in gas cycle
refrigeration systems. This may be thought of as a modification of reversed Carnot cycle, as the
two isothermal processes of Carnot cycle are replaced by two isobaric heat transfer
processes. This cycle is also called as Joule or Bell-Coleman cycle. Figure 9.2(a) and(b) shows the schematic of a closed, reverse Brayton cycle and also
the cycle on T-s diagram. As shown in the figure, the ideal cycle consists of the
following four processes:
Process 1-2: Reversible, adiabatic compression in a compressor Process
2-3: Reversible, isobaric heat rejection in a heat exchanger Process
3-4:
Reversible, adiabatic expansion in a turbine
Process 4-1 : Reversible, isobaric heat absorption in a heat exchanger
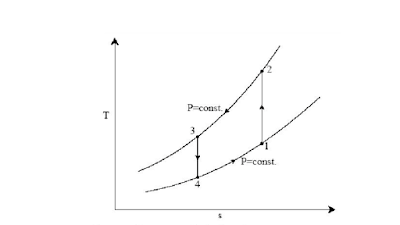 | Reverse Brayton cycle in T-s plane
|
Process 1-2:
Gas at low pressure is compressed isentropically from state 1 to state 2. Applying
steady flow energy equation and neglecting changes in kinetic and potential energy, we
can write:
Process 2-3:
Hot and high pressure gas flows through a heat exchanger and rejects heat sensibly and
isobarically to a heat sink. The enthalpy and temperature of the gas drop during the
process due to heat exchange, no work transfer takes place and the entropy of the gas
decreases. Again applying steady flow energy equation and second T ds equation:
Process 3-4:
High pressure gas from the heat exchanger flows through a turbine, undergoes isentropic
expansion and delivers net work output. The temperature of the gas drops during the process
from T3 to T4. From steady flow energy equation:
Process 4-1:
Cold and low pressure gas from turbine flows through the low temperature
heat exchanger and extracts heat sensibly and isobarically from a heat source,
providing a useful refrigeration effect. The enthalpy and temperature of the gas rise
during the process due to heat exchange, no work transfer takes place and the entropy of
the gas increases. Again applying steady flow energy equation and second T ds
equation:
Comparison of reverse Carnot and
reverse Brayton cycle in T-s plane
a) COP of
Brayton cycle approaches COP of Carnot cycle as T 1 approaches T4 (thin
cycle), however, the specific refrigeration effect [cp(T1-T4)] also reduces simultaneously.
b) COP of
reverse Brayton cycle decreases as the pressure ratio rp increases
Actual reverse Brayton cycle:
The actual
reverse Brayton cycle differs from the ideal cycle due to:
i. Non-isentropic compression and expansion
processes
ii. Pressure drops in cold and hot heat exchangers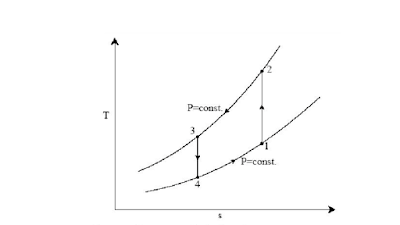
Comparison of ideal and actual Brayton cycles T-s plane
Due to these irreversibilities, the
compressor work input increases and turbine work output reduces. thus the net
work input increases due to increase in compressor work input and reduction in turbine
work output. The refrigeration effect also reduces due to the
irreversibilities. As a result,
the COP of actual reverse Brayton cycles will be considerably lower than the
ideal cycles.
Design of efficient compressors and turbines plays a major role in improving
the COP of the
system. In practice,
reverse Brayton cycles can be open or closed. In open systems, cold air at the exit of the
turbine flows into a room or cabin (cold space), and air to the compressor is taken from
the cold space. In such a case, the low side pressure will be atmospheric. In closed
systems, the same gas (air) flows through the cycle in a closed manner. In such cases it is
possible to have low side pressures greater than atmospheric. These systems are known as
dense air systems. Dense air systems are advantageous as it is possible to
reduce the volume
of air handled by the compressor and turbine at high pressures. Efficiency will also be high
due to smaller pressure ratios. It is also possible to use gases other than air (e.g.
helium) in closed systems.
|
0 Comments:
Post a Comment
Thank you for the comment